Genome editing, also called gene editing, is a cluster of technologies allowing scientists the ability to change an organism’s DNA.
The technologies around gene editing allow genetic material to be added, removed, or altered at particular locations in the genome. Several approaches to genome editing have been developed. A recent one is known as CRISPR-Cas9, or clustered regularly interspaced short palindromic repeats, and CRISPR-associated protein 9. The CRISPR-Cas9 system has generated a lot of excitement in the scientific community because it is faster, cheaper, more accurate, and more efficient than other existing genome editing methods.
CRISPR-Cas9 was adapted from a naturally occurring genome editing system in bacteria. The bacteria capture snippets of DNA from invading viruses and use them to create DNA segments known as CRISPR arrays. The CRISPR arrays allow the bacteria to “remember” the viruses or closely related ones. If the viruses attack again, the bacteria produce RNA segments from the CRISPR arrays to target the viruses’ DNA. The bacteria then use Cas9 or a similar enzyme to cut the DNA apart, which disables the virus.
The CRISPR-Cas9 system works similarly in the lab. Researchers create a small piece of RNA with a short “guide” sequence that attaches (binds) to a specific target sequence of DNA in a genome. The RNA also binds to the Cas9 enzyme. As in bacteria, the modified RNA is used to recognize the DNA sequence, and the Cas9 enzyme cuts the DNA at the targeted location. Although Cas9 is the enzyme that is used most often, other enzymes (for example Cpf1) can also be used. Once the DNA is cut, researchers use the cell’s own DNA repair machinery to add or delete pieces of genetic material, or to make changes to the DNA by replacing an existing segment with a customized DNA sequence.
Genome editing is of great interest in the prevention and treatment of human diseases. Currently, most research on genome editing is done to understand diseases using cells and animal models. Scientists are still working to determine whether this approach is safe and effective for use in people. It is being explored in research on a wide variety of diseases, including single-gene disorders such as cystic fibrosis, hemophilia, and sickle cell disease. It also holds promise for the treatment and prevention of more complex diseases, such as cancer, heart disease, mental illness, and human immunodeficiency virus (HIV) infection.
Ethical concerns arise when genome editing, using technologies such as CRISPR-Cas9, is used to alter human genomes. Most of the changes introduced with genome editing are limited to somatic cells, which are cells other than egg and sperm cells. These changes affect only certain tissues and are not passed from one generation to the next. However, changes made to genes in egg or sperm cells (germline cells) or in the genes of an embryo could be passed to future generations. Germline cell and embryo genome editing bring up a number of ethical challenges, including whether it would be permissible to use this technology to enhance normal human traits (such as height or intelligence). Based on concerns about ethics and safety, germline cell and embryo genome editing are currently illegal in many countries.
Patent Dispute
Last year, the Patent Trial and Appeal Board (PTAB) of the U.S. Patent and Trademark Office (USPTO) declared an interference between 10 University of California (UC) patent applications and multiple previously issued Broad Institute patents, according to UC-Berkeley.
The action jeopardizes 13 of the Broad’s 15 CRISPR-Cas9 U.S. patents and one patent application, and signals that the USPTO will take up the issue of who first invented CRISPR-Cas9 genome editing in eukaryotic cells, that is, plant and animal cells.
The CRISPR-Cas9 DNA-targeting technology was invented by Jennifer Doudna and Martin Jinek at the University of California, Berkeley; Emmanuelle Charpentier, then of Umea University in Sweden; and Krzystof Chylinski at the University of Vienna.
The declaration of interference affects 13 of the Broad’s 15 U.S. patents, as well as one application — essentially all of its CRISPR patents involving eukaryotic cells. The six U.S. patents received to date by UC are not included in this interference. Based on current PTAB interference schedule, the interference is likely to be completed within two years.
CRISPR’s Future
In standard CRISPR, a scissor-like enzyme called Cas9 is used to cut all the way through both strands of the DNA molecule’s double helix. That usually results in the cell’s DNA repair apparatus inserting or deleting DNA letters at the site. As a result, CRISPR is extremely useful for disrupting genes and inserting or removing large DNA segments. However, it is difficult to use this system to make more subtle corrections to DNA, such as swapping a letter T for an A.
To expand the gene-editing toolbox, a research team led by David R. Liu, Broad Institute of MIT and Harvard, Cambridge, MA, previously developed a class of editing agents called base editors [2,3]. Instead of cutting DNA, base editors directly convert one DNA letter to another. However, base editing has limitations, too. It works well for correcting four of the most common single letter mutations in DNA. But at least so far, base editors haven’t been able to make eight other single letter changes, or fix extra or missing DNA letters.
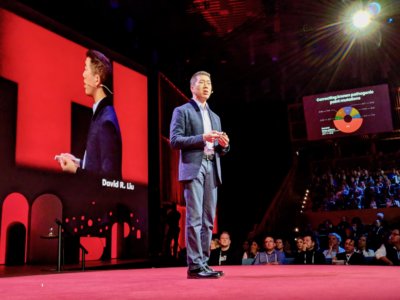
In contrast, the new prime editing system can precisely and efficiently swap any single letter of DNA for any other, and can make both deletions and insertions, at least up to a certain size. The system consists of a modified version of the Cas9 enzyme fused with another enzyme, called reverse transcriptase, and a specially engineered guide RNA, called pegRNA. The latter contains the desired gene edit and steers the needed editing apparatus to a specific site in a cell’s DNA.
Once at the site, the Cas9 nicks one strand of the double helix. Then, reverse transcriptase uses one DNA strand to “prime,” or initiate, the letter-by-letter transfer of new genetic information encoded in the pegRNA into the nicked spot, much like the search-and-replace function of word processing software. The process is then wrapped up when the prime editing system prompts the cell to make the other DNA strand to match the new genetic information.